Sea turtle ocean highways tracked through satellite telemetry and the NEMO ocean model.
Many marine organisms travel great distances which presents a variety of research and conservation challenges. The movements of large animals like sharks, marine mammals and sea turtles are now routinely studied with satellite tracking technology. Once attached to an animal, these satellite tracking devices send us regular data on the animal’s location. For sea turtles, satellite tracking devices can easily be attached to adults when they come ashore to nest. Consequently, the regular migrations between their breeding and foraging habitats (which can be several 100s to 1000s of kilometres apart) are now well documented. However, one of the biggest challenges in satellite tracking technology is the miniaturisation of these devices. Consequently, many small marine species and juveniles of larger species are too small to be tracked in this way. This is the case for new born hatchling sea turtles which are just a few centimetres when they emerge from their nests; much smaller than the satellite tags currently available. It is thus very difficult to study hatchling sea turtles during their first few years of life (which are commonly referred to as the “lost years”) as these small hatchlings disappear into the sea after emerging from their nests.
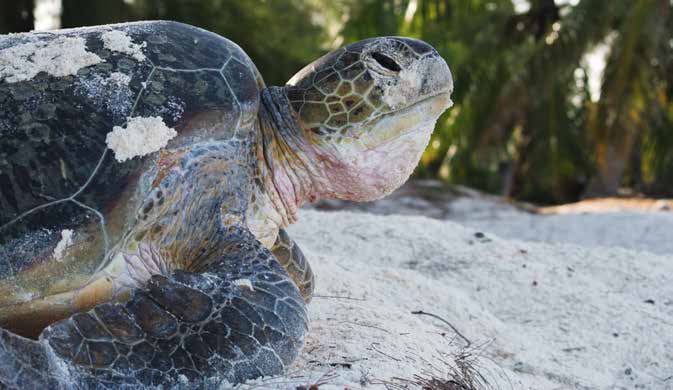
Nonetheless, it is now well established that surface ocean currents drive the dispersion of hatchling turtles from predator-rich nesting beaches to safer oceanic habitats where they live and grow as juveniles. Indeed, ocean currents can transport hatchlings on journeys that can span entire ocean basins; hence the distances that they travel can be much further than the regular breeding migrations of adult turtles. In the North Atlantic for example, loggerhead turtle hatchlings from nesting beaches in the southeastern USA are transported north with the Gulf Stream current and then east with currents at the northern boundary of a large clockwise flowing ocean circulation system, the North Atlantic gyre. Transported in this gyre, hatchlings are able to reach their oceanic development habitats near the Azores islands > 5000 kms away from where they were born. Hatchlings then remain at the Azores for several years before they return to coastal habitats in the southeastern USA as large juvenile turtles. During their return journeys they are aided by the westerly flowing currents at the southern boundary of the North Atlantic gyre. These large juvenile turtles then remain in these coastal habitats until they reach maturity and return to the area where they were born to breed. Whilst hatchlings are too small to be directly tagged/followed on these long journeys, oceanography approaches can be used to study hatchlings during the “lost years”.
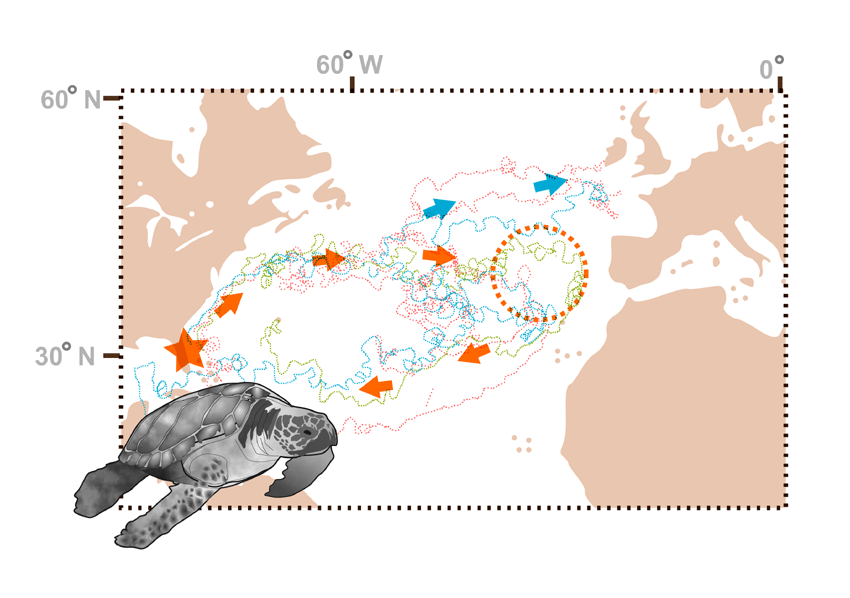
Measurements of ocean currents are commonly made by releasing objects e.g. surface drifter buoys, into the ocean and tracking their movement pathways. Thousands of satellite tracked surface drifter buoys have now been deployed throughout the oceans as part of the Global Drifter Programme (GDP). Data from the GDP is freely available online and is a very easy source of information for marine biologists to work with. Additionally, sophisticated global ocean circulation models such as the “NEMO” ocean model can be valuable tools for biologists as they enable us to release “virtual floating objects” anywhere in the ocean and the movements of these virtual floats can be tracked on a computer programme as they drift in modelled ocean current simulations. Hence, for small organisms that disperse with ocean currents, drifter buoys and ocean models like NEMO can be used in innovative ways by biologists wanting to study these cryptic animals.
For example, from beaches in the southeastern USA, the loggerhead turtle hatchlings that disperse in the North Atlantic gyre are typically not seen again until they are encountered by fisherman in the Azores. By using drifter buoys and ocean models to study the ocean current flows (and therefore dispersal pathways of hatchlings) between the southeastern USA nesting beaches and juvenile habitats in the Azores, we can get important information about the “lost years”. Due to the small size of hatchlings and the huge distances that they disperse, obtaining even very basic information on the biology of hatchlings is difficult. However, by combining data on (1) the size of new born hatchlings when they first emerge from their nests with (2) data on the size of hatchlings that are accidentally caught by fisherman in the Azores with (3) the time we estimated that it takes for hatchlings to drift between these sites, we were able to produce the first robust estimates of the growth rates of hatchlings.
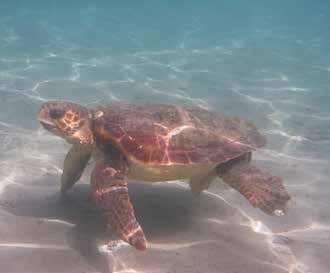
Unlike fish and other marine organisms, for which there are well established techniques to directly determine the age of individual animals and hence the age at which species reach maturity, sea turtles cannot be aged directly with accuracy. Instead, reliable age estimates require information on the natural growth rates of hatchling turtles, larger juvenile turtles and adult turtles. The growth rates of large juvenile and adult turtles can be measured at the breeding grounds and in coastal foraging habitats through mark-recapture programmes where turtles can easily be captured, measured and marked (typically with a metal flipper tag), and then recaptured/measured at a later date. Prior to our estimate, however, the growth rates of hatchling turtles were only well known for hatchlings in captivity. Since these captive individuals are given a lot more food than they would get naturally in the wild, captive growth rates are much higher than natural growth rates. So, by combining our new hatchling growth rate estimates during the “lost years” with direct growth measurements of larger turtles, we were able to produce the most reliable age at maturity estimate to date. In so doing, we revealed that the time these turtles take to reach maturity (c. 45 years) is much longer than other animal species and that past age at maturity estimates based on captive growth rates were underestimated. This has important conservation implications as species, like turtles, which take such a long time to reach maturity, are much more vulnerable to extinction than other species (like fish, marine mammals, birds, lizards etc) that mature at much younger ages.
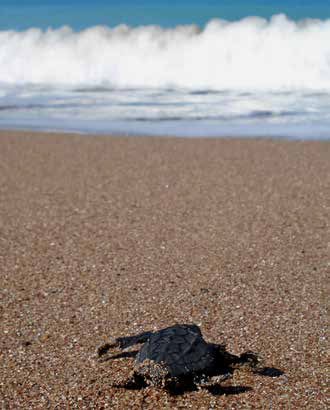
Since hatchlings are reliant on ocean currents to reach their oceanic development habitats, we can use oceanography data to study the “lost years” and gain important information on sea turtle biology. However, laboratory observations by American researchers have also shown that hatchlings can undertake in periods of active swimming. For example, when they first enter the sea they embark on a period of intense offshore swimming (the “swimming frenzy”) which lasts for about a week. This frenzy period helps hatchlings escape predator-rich coastal waters and reach offshore currents like the Gulf Stream which rapidly transports them towards safer oceanic habitats near to the Azores. Once offshore, hatchlings are also known to be able to use geomagnetic information from the Earth’s magnetic field as a navigational sign post. By adding laboratory observations of this swimming behaviour into ocean models we were able to show for the first time that limited amounts of swimming (just 1-3 hrs/day) can (1) help hatchlings to remain within the warm waters of the Gyre/reach safe offshore development habitats like the Azores and (2) avoid drifting towards northern Europe where the cold water would kill them. This is quite remarkable given the limited swimming capabilities of hatchlings (due to their small size) and the fact that these simulations were carried out in a region that contains one of the fastest current flows in the World (the Gulf Stream).
Our work highlights the value of oceanography approaches for studying the biology of species which are difficult to study using conventional techniques and the importance of taking into account the behaviour of animals when using these valuable, albeit currently underexploited, oceanographic tools. Many other marine species, like fish, have juvenile life stages that also disperse with ocean currents and the swimming speed and strength of these juveniles are comparable or greater than those of hatchling sea turtles. The potential conservation applications of these oceanographic tools are thus huge as key information can be gained on the pathways, habitats, survival and biology of a range of species of conservation concern. This information is all crucial for designing effective conservation and management strategies.